Improving the blast resilience of rail vehicles
- Like
- Digg
- Del
- Tumblr
- VKontakte
- Buffer
- Love This
- Odnoklassniki
- Meneame
- Blogger
- Amazon
- Yahoo Mail
- Gmail
- AOL
- Newsvine
- HackerNews
- Evernote
- MySpace
- Mail.ru
- Viadeo
- Line
- Comments
- Yummly
- SMS
- Viber
- Telegram
- Subscribe
- Skype
- Facebook Messenger
- Kakao
- LiveJournal
- Yammer
- Edgar
- Fintel
- Mix
- Instapaper
- Copy Link
Posted: 3 March 2014 | Jean-Luc Bruyelle, El-Miloudi El-Koursi, Amaury Flancquart, IFFSTAR Richard Seddon, Tecnalia; Conor O'Neill, NewRail; Antonio De Santiago Laport, Metro de Madrid | No comments yet
In recent years, terrorist attacks on metro systems have noticeably increased, as illustrated for instance by the Paris, Madrid, and London attacks. These dramatic events have raised concern regarding the ability to increase the efficiency of the management of similar crises in order to reduce the number and gravity of the victims, as well as easing the organisational efficiency in order to resume service quickly and avoid societal disruption. Indeed, several efforts aim at improving the resilience of the metro coaches to achieve the following three main goals…
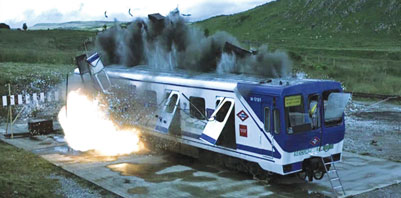
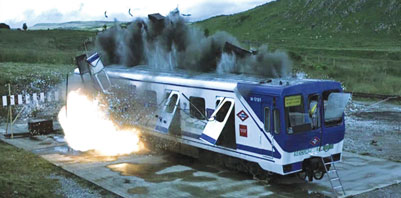
High speed image of the trial with an existing Metro de Madrid carriage
- Increase the resilience of vehicles to blast through the selection of vehicle materials and structural design in order to speed-up the recovery following an attack, allowing the transport system to return to normal operation quickly
- Increase the security against a firebomb attack through fire barriers and fire suppression, while also contributing to passenger safety from accidental or vandalism fires
- Reduce the attractiveness of metro systems as a target for attack by reducing the human, economic and organisational impacts of such attacks.
Such efforts include the European-funded FP7 SECUREMETRO project, which reached its conclusion in June 2013, and gathered the following 11 partners from the United Kingdom, Spain, France and Italy:
- Academic and applied research: Newrail (UK), Tecnalia (Spain), IFSTTAR (France), Istituto Afari Internazionali (Italy), and the Spanish Railways Foundation (Spain)
- Underground operators: Metro de Madrid (Spain) and RATP (France)
- Industry: Bombardier (France), Maxam- Expal (Spain), Sunsundegui (Spain) and STAM (Italy).
SECUREMETRO aimed at bringing solutions to mitigate the consequences of an internal blast in metro vehicles. It adds up to more conventional approaches to reduce the casualties and disruptions related to terrorist attacks, although its findings are also relevant to accidental events. Among the efforts dedicated to the terrorist threat, SECUREMETRO is original in that it does not strive to mitigate the possibility of an attack, but its consequences. These goals are achieved by using validated materials and design strategies to build metro vehicles with intrinsic security features2.
Another important consideration is that the SECUREMETRO solutions are designed to be implementable as a retrofit for the existing rolling stock. This both helps keeping the implementation costs at an affordable level, and reducing the delay to introduce the improvement since most existing rolling stock will not be replaced for decades.
Terrorist threat on metro vehicles and its evolution
The first step was an assessment of past attacks in order to identify the most likely and most harmful types of attack. This analysis used the Database of Worldwide Terrorism Incidents of the Rand Corporation and the University of Maryland’s Global Terrorism Database.
Over the last decades, the number of terrorist attacks on rail-based transportation has evolved in considerable proportions. During the 1960-2010 period, 833 attacks killed approximately 3,500 people and injured more than 15,000. Moreover, figures for both attacks and victims increased sharply around the 1980s (see Figure 1).
The perpetration tactic is of particular importance to devise ways to mitigate the effects of the attack. Out of the 833 attacks, bombs were used as a weapon in 73% of the cases, followed by firearms (6%), firebombs (4%), and fires (4%). The other types of weapons are marginal. These proportions have not significantly evolved during the last decade.
This is confirmed by the number of victims, with 70% of the fatalities (2,541 out of 3,457) and 77% of the injuries (7,832 out of 10,6823) caused by bombings during the considered period, making it the deadliest type of attack. The trend remains stable in the recent period, which leads to expect a continuing increase of the number of attacks and victims, with a vast majority of bombings.
Findings from the analysis of existing attacks
SECUREMETRO concentrated on specific cases chosen for their representativeness and the abundance and relevance of the documentation. These cases are notably the bomb attacks in London (2005) and Madrid (2004), and fires in the Daegu Metro (2003) and the Kaprun Funicular (2001). Other related situations, such as 9/11 or fires in high buildings and other places from which evacuation is difficult, were also considered. The project found considerable insight in the previous work carried out in these fields4,5,6.
An explosion causes damage through several mechanisms, causing specific types of disruptions and calling for different mitigation measures. The primary effects are the shockwave and the blast, as well as primary fragments from the bomb itself: pieces of its case and artefacts such as nails. These effects are inherent to the bomb and can only be alleviated by shielding or dampening measures to dissipate the blast and to prevent the propagation of fragments. Another effect is the creation of secondary fragments, caused by fragmentation of the inner structures, walls or windows, turned into shrapnel. The last mechanical effect is the loss of structural integrity, causing collapse of overhead equipment or of the roof. Although it is impossible to design a 100% infallible protection system, it is possible to greatly improve the protection of the passengers from falling debris which is a major cause of head injuries.
It was found that non-incendiary bombs do not usually cause a fire, and that the current EN45545 standard is adequate to prevent fire in these conditions.
Although the inner structure of the vehicle was mostly destroyed in the studied cases, the overall structure, wheels, bogies and floor, was mostly intact, allowing access and even towing away. This observation, that holds true even with high explosive loads, motivated us to consider resilience, both from the technical and organisational points-of-view: if a bombed vehicle has surviving passengers and is still able to operate, even in a very limited manner, it is desirable to add resilience in the design to ease and speed-up the rescue and the recovery of the system after the blast.
Designs improvements
Particular attention was paid to specific key systems and components of the metro vehicle that must be considered for the safety of the passengers on a bombed train:
Windows/glazing
Windows and glazing should be able to deform plastically, absorbing part of the energy of the blast and minimising the fragments. High resilience and high plasticity materials should be employed to avoid glass shards. Appropriately supported laminated glass panels have far superior properties that allow them to absorb energy with little or no fragmentation. Another important element is the support system: particular attention was paid to the connection between the window and the frame, and between the frame and the carriage structure.
Walls
Walls should be able to deform plastically without fragmenting or breaking into large pieces that could be projected inside by the negative pressure following the blast wave.
External doors
Current rolling stock metro trains have sliding doors, whose mechanism can be severely affected by a blast. External doors should not be thrown away.
Interlocking doors
Results from the RAILPROTECT project show that internal walls can contain a blast, limiting its effect inside the carriage. Modern trains without interlocking doors can be improved using transparent transversal walls.
Roof
The roof breaks into large pieces under the effect of the blast wave. The roof panels should not fall inside the carriage and injure the passengers. Mechanical systems, like tethered cables, should prevent the panels to be thrown inside.
Seats
The most important issue concerning seating is their connection with the vehicle structure, because the blast wave could tear them away from the floor.
HVAC
HVAC and other heavy equipment are typically located on the roof of the carriage. As a result they can fall inside the vehicle and hurt the passengers. They would be best placed under the floor, protected by the strong bogies structures.
Critical systems
Since the floor is little affected except at the location of the explosion, all the main supply and critical systems may be protected by placing wires, pipes, etc., inside steel/aluminium cases and ducts placed beneath the floor. A survivable driver’s cab could allow driving the train to the closest station, which would dramatically ease rescue and evacuation. All this would increase the weight of the train, so attention should be paid to the mass of the carriage components and to the materials used.
Cost considerations
The modifications required to make a metro train blast safe could require a very high investment, which not everyone can afford. A possible solution is a sort of ‘blast-resilient certification’ for metro lines. Cities where the risk of metro bombings is low may not be interested in a large investment for blast resistant trains. On the contrary, medium/high risk cities like London, Paris and Madrid could be interested in being certified as ‘blast resilient’.
Experimental assessment
A full-size blast test was performed to assess the performance of current rolling stock. This test was carried out on a decommissioned Series 5000 carriage given to the SECUREMETRO project by Metro de Madrid. This type of carriage is typical of the rolling stock built in the mid-70s and still in operation today. The trial consisted of detonating a charge similar to those used in the London attacks, located at the centreline of the carriage.
As shown in Figure 2, the two doors on both sides of the carriage, at the explosion point, became detached and landed 60m away. Several other passenger doors were removed from the carriage, while others were buckled and inoperable. All the windows on the right side within the passenger area were shattered and their rubber seals dislodged from their frames. In the driver’s cab, all the windows and doors were dislodged, except the access door which remained operable.
However, damage to the chassis was limited to the rear heat exchanger pack being broken from its mounts, while the central pack was only broken at its rear point. The air reservoir remained intact. Overall, the chassis fared well and the carriage could be towed away, confirming that improved resilience in the passenger area would dramatically improve the security in case of blast.
Analysis of the effects, based on this test and the case studies, identified several key areas for improvement, mainly: windows; doors; interlocking doors; components materials; joining techniques; information and communication systems; and evacuation system.
Computer simulation was performed to better understand the behaviour of the shock wave inside the carriage, identify the most stressed parts of the internal structure, and devise and select structural improvements to improve resilience. This led to a series of small-scale blast tests of unitary samples to assess the improvement over existing materials.
The final step then consisted of integrating these solutions in a demonstrator vehicle, and submitting it to the same blast test as the existing vehicle. The improvements brought to the demonstrator consisted notably of:
- Improved resistance of the windows that cleanly separate from the body and do not shatter, thanks to protective film and bonding
- Improved resistance of the ceiling panels and ceiling-mounted elements using retaining cables to the main vehicle structure: the ceiling does not fall on the passengers, and does not cover the ground with debris which would make walking difficult and hazardous
- Reinforced lighting using LEDs
- Reinforced driver’s bulkhead
- Use of flexible backing layer (polyurea) on key elements of the secondary structure to improve flexibility and avoid fragmentation.
As show in Figure 3, in the blast test the driver’s bulkhead failed, which the simulation had not predicted. However, the pressure data close to the bulkhead allowed to determine the reasons of the failure, and to propose the necessary reinforcement.
The floor was punctured below the blast point but did not cause structural failure. Several window bonding solutions were simultaneously tried, and none shattered except the unprotected (reference) window which failed as expected. All the bonded windows stayed in place. The emergency window, protected by film but not bonded to the structure, was ejected in one piece: leaving the frame open but free from glass fragments, allowing safe egress.
The seating remained mostly intact, except the seats closest to the charge which were fractured but did not generate secondary fragments.
The ceiling panels attached by cables to the main vehicle structure remained attached and did not fall, making walking in the carriage much easier and safer than in the unprotected carriage. Panels attached to secondary elements fell to the floor, showing the importance of tying to the main vehicle structure.
The prototype LED lighting worked throughout and also after the trial.
Overall, these results confirmed the improvement to the resilience of the metro vehicle, passengers and staff. Another achievement of SECUREMETRO is the design of a testing set-up that was an improvement over that used during the first trial.
Conclusion
The outcome of the project is expected to yield improvements in the design of metro vehicles to improve blast resilience, and their testing led to the design of measurement methodologies suitable for the specific context of an explosion inside a carriage. It therefore appears that the raised issues, and the solutions found, are both worthy of consideration by the standardisation bodies.
Acknowledgements
The authors would like to thank the European Commission for supporting the funding for the ‘SECUREMETRO – Inherently secure blast resistant and fire safe metro vehicles’ project (Grant Agreement No. 234148), and also wish to thank all the partners of the SECUREMETRO project.
References
- http://securemetro.inrets.fr
- ‘Improving the resilience of metro vehicles and passengers for an effective emergency response to terrorist attacks’ (J.-L. Bruyelle, C. O’Neill, E.-M. El-Koursi, F. Hamelin, N.Sartori), Safety Science Journal, Safety Science, n° 62 (2014), pp. 37-45; http://www.sciencedirect.com/science/article/pii/S0925753513001720
- Excluding the five fatalities and 5,205 casualties in the 1995 Tokyo gas attack which remains the only one of its kind.
- ‘The mass psychology of disasters and emergency evacuations: a research report and implications for practice’ (J. Drury and C. Cocking, 2007); http://www.sussex.ac.uk/affiliates/panic/Disasters%20and%20emergenc y%20evacuations%20(2007).pdf
- ‘To prevent ‘panic’ in an underground emergency: why not tell people the truth?’ (G. Proulx and J.D. Sime), Proc. 3rd symposium on fire safety science, pp. 843-852. Elsevier Applied Science, London, 1991.
- ‘The nature of collective resilience: survivor reactions to the 2005 London bombings’ (J. Drury, C. Cocking and S. Reicher) Int. Journal of Mass Emergency and Disasters, vol. 27, no. 1, pp. 66-95., 2009.
Biography
Jean-Luc Bruyelle received a PhD in Image-Processing in 1994, and since then has been a Researcher in the domain of transportation at the University of Lille, the Catholic University of Louvain and IFSTTAR. His main fields of expertise are safety, image-processing and assessment. Jean-Luc has participated and coordinated work packages in ANR and European FP and Eureka projects, including PROMETHEUS, CROMATICA, PRISMATICA, BOSS, PANSAFER, RESTRAIL and SECUREMETRO.
El-Miloudi El-Koursi is a Research Director at IFSTTAR, with 30 years of experience in the field of railways. His domains are safety, control/command, security and assessment.
Richard Seddon is a Project Manager at Tecnalia Research and Innovation. He has coordinated work packages and participated in several European research projects focused on the development of composite and hybrid materials for extreme conditions (EXTREMAT, KMM, AGAPAC, ADVITAC, ELECTRICAL and SECUREMETRO). Richard also acts as a technology transfer broker for the European Space Agency.
Conor O’Neill joined NewRail as a Research Engineer focusing on light-weight materials and structures. An aeronautical engineering graduate of the University of Limerick in Ireland, Conor has focused his career on the manufacture and testing of composite materials. He worked at Airbus for seven years in the Composite R&D and Military Transport Aircraft Test Departments. Conor is the Coordinator of two European FP7 projects – SecureMetro and FireResist.
Amaury Flancquart is an Engineer at the LEOST lab, IFSTTAR. His main work is related to the design and development of hardware and software architectures for audio and video perception and positioning systems. He has been involved in several experimentations in European research projects (PRISMATICA, LOCOPROL, BOSS, SATLOC and SECUREMETRO).
Antonio De Santiago Laporte is an Industrial Technical Engineer. After more than 12 years working for AT&T Microelectronics, Lucent Technologies Microelectronics Group and Agere Systems, he finally joined Metro de Madrid in 2002. Initially involved in the Fixed Installations Maintenance, Antonio has been working since 2007 in the different R&D Departments of the company, actually as the R&D Coordinator, dealing with the National and European projects in which Metro de Madrid is participating (VI and VII Framework programs, Horizon2020).
Related topics
Security & Crime, Vehicle & Passenger Safety
Issue
Issue 1 2014
Related modes
Metro
Related organisations
Metro de Madrid, NewRail
Related people
Conor O’Neill